The Double-beam UV-visible Absorption Spectrometer
This page describes a double-beam UV-visible absorption spectrometer in enough detail to meet the requirements of UK-based chemistry courses for 16 – 18 year olds.
The Overall Design
The Aims
If you pass white light through a coloured substance, some of the light gets absorbed. A solution containing hydrated copper(II) ions, for example, looks pale blue because the solution absorbs light from the red end of the spectrum. The remaining wavelengths in the light combine in the eye and brain to give the appearance of cyan (pale blue).
Some colourless substances also absorb light – but in the ultra-violet region. Since we can't see UV light, we don't notice this absorption.
Different substances absorb different wavelengths of light, and this can be used to help to identify the substance – the presence of particular metal ions, for example, or of particular functional groups in organic compounds.
The amount of absorption is also dependent on the concentration of the substance if it is in solution. Measurement of the amount of absorption can be used to find concentrations of very dilute solutions.
An absorption spectrometer measures the way that the light absorbed by a compound varies across the UV and visible spectrum.
A Simple Double-beam Spectrometer
We'll start with the full diagram, and then explain exactly what is going on at each stage.
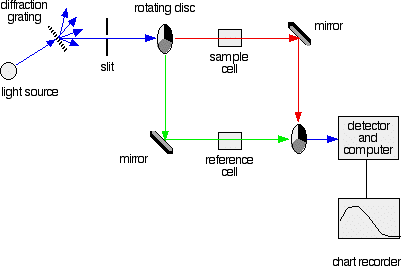
IMPORTANT! The colour-coding of the light beams through the spectrometer is NOT to show that some light is red or blue or green. The colours are simply to emphasise the two different paths that light can take through the device. Where the light is shown as a blue line, this is the path that it will always take. Where it is shown red or green, it will go either one way or the other – depending on how it strikes the rotating disc (see below).
Explaining What's Happening
The Light Source
You need a light source which gives the entire visible spectrum plus the near ultra-violet so that you are covering the range from about 200 nm to about 800 nm. (This extends slightly into the near infra-red as well.)
Note: "Near UV" and "near IR" simply means the parts of the UV and IR spectra which are close to the visible spectrum. If you aren't happy about how the various types of electromagnetic radiation relate to each other, follow this link before you go on.
You can't get this range of wavelengths from a single lamp, and so a combination of two is used – a deuterium lamp for the UV part of the spectrum, and a tungsten / halogen lamp for the visible part.
Note: A deuterium lamp contains deuterium gas under low pressure subjected to a high voltage. It produces a continuous spectrum in the part of the UV spectrum we are interested in.
The combined output of these two bulbs is focussed on to a diffraction grating.
The Diffraction Grating and the Slit
You are probably familiar with the way that a prism splits light into its component colours. A diffraction grating does the same job, but more efficiently.
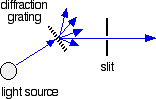
The blue arrows show the way the various wavelengths of the light are sent off in different directions. The slit only allows light of a very narrow range of wavelengths through into the rest of the spectrometer.
By gradually rotating the diffraction grating, you can allow light from the whole spectrum (a tiny part of the range at a time) through into the rest of the instrument.
The Rotating Discs
This is the clever bit! Each disc is made up of a number of different segments. Those in the machine we are describing have three different sections – other designs may have a different number.
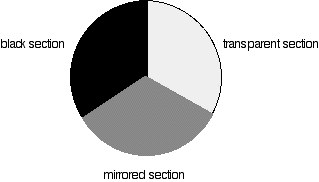
The light coming from the diffraction grating and slit will hit the rotating disc and one of three things can happen.
- If it hits the transparent section, it will go straight through and pass through the cell containing the sample. It is then bounced by a mirror onto a second rotating disc.
This disc is rotating such that when the light arrives from the first disc, it meets the mirrored section of the second disc. That bounces it onto the detector.
It is following the red path in the diagram: - If the original beam of light from the slit hits the mirrored section of the first rotating disc, it is bounced down along the green path. After the mirror, it passes through a reference cell (more about that later).
Finally the light gets to the second disc which is rotating in such a way that it meets the transparent section. It goes straight through to the detector. - If the light meets the first disc at the black section, it is blocked – and for a very short while no light passes through the spectrometer. This just allows the computer to make allowance for any current generated by the detector in the absence of any light.


The Sample and Reference Cells
These are small rectangular glass or quartz containers. They are often designed so that the light beam travels a distance of 1 cm through the contents.
The sample cell contains a solution of the substance you are testing – usually very dilute. The solvent is chosen so that it doesn't absorb any significant amount of light in the wavelength range we are interested in (200 – 800 nm).
The reference cell just contains the pure solvent.
The Detector and Computer
The detector converts the incoming light into a current. The higher the current, the greater the intensity of the light.
For each wavelength of light passing through the spectrometer, the intensity of the light passing through the reference cell is measured. This is usually referred to as I0 – that's I for Intensity.
The intensity of the light passing through the sample cell is also measured for that wavelength – given the symbol, I.
If I is less than I0, then obviously the sample has absorbed some of the light. A simple bit of maths is then done in the computer to convert this into something called the absorbance of the sample – given the symbol, A.
For reasons which will become clearer when we do a bit of theory on another page, the relationship between A and the two intensities is given by:
On most of the diagrams you will come across, the absorbance ranges from 0 to 1, but it can go higher than that.
An absorbance of 0 at some wavelength means that no light of that particular wavelength has been absorbed. The intensities of the sample and reference beam are both the same, so the ratio I0/I is 1. log10 of 1 is zero.
An absorbance of 1 happens when 90% of the light at that wavelength has been absorbed – which means that the intensity is 10% of what it would otherwise be.
In that case, I0/I = 100 / 10 = 10 and log10 of 10 is 1.
Note: If you don't feel comfortable with logarithms, don't worry about it. Just accept that an absorbance scale often runs from zero to 1, but could go higher than that in extreme cases (in other words where more than 90% of a wavelength of light is absorbed).
The Chart Recorder
Chart recorders usually plot absorbance against wavelength. The output might look like this:

This particular substance has what are known as absorbance peaks at 255 and 395 nm. How these arise and how they are interpreted are discussed on another page.
Where would you like to go now?
To the UV-visible spectroscopy menu To the analysis menu To Main Menu